Prevention of Infections Due to Antibiotic-Resistant Bacteria
Marin H. Kollef, MD, FCCP &
Kenneth V. Leeper, Jr, MD
Copyright © 2005 MedScholar.
Introduction
Antimicrobial resistance
among bacterial pathogens has become a serious health threat, and the
incidence is rising at an alarming rate. Every year, nearly 2 million
patients in American hospitals acquire a nosocomial infection, and
more than 70% of the causative pathogens are resistant to at least one
of the antibiotics most commonly used to treat them.1 Over 50% of
Staphylococcus aureus isolates in intensive care units (ICUs) are
resistant to methicillin, oxacillin, or nafcillin (MRSA); 25% of
enterococcal isolates are resistant to vancomycin; and 87% of
coagulase-negative staphylococci are resistant to methicillin.2 An
increasing rate of antibiotic-resistant gram-negative bacilli also has
been reported, with the highest incidence seen in Pseudomonas
aeruginosa.3 The rise of MRSA has been particularly precipitous and
now accounts for about 40% of all nosocomial S aureus infections in
the United States.4
Resistant pathogens have
been associated with an increased rate of in-hospital mortality,
increased morbidity, and a longer length of hospital stay.5 Patients
with infections caused by resistant bacteria also are more likely to
receive inadequate antimicrobial treatment and incur higher healthcare
costs relative to those with antibiotic-susceptible bacterial
infections. In US hospitals, annual estimated expenses associated with
antibiotic resistance range from $100 million to $30 billion.4
Multiple factors
contribute to the escalating emergence of antibiotic resistance. These
include the inappropriate use and misuse of antibiotics, especially
frequent and unnecessary use of broad-spectrum agents; increasing use
of invasive devices; reductions in nursing and other support staff,
which raises the likelihood of person-to-person transmission of
microorganisms; and increasing numbers of patients with
immunosuppression and other similar conditions who are at higher risk
for acquiring a drug-resistant infection.6 In addition, prolonged
hospitalization appears to predispose patients to either infection or
colonization with resistant bacteria.
Preventing the
Emergence of Resistance
Although resistance is not
a new phenomenon, the incidence has increased dramatically over the
past 2 decades. The development of new drugs has slowed considerably
and may be unable to keep pace with the continuing growth of pathogen
resistance. Therefore, effective strategies are needed to prevent the
continuing emergence of antimicrobial resistance. These include the
avoidance of unnecessary antibiotic administration and increasing the
effectiveness of prescribed antibiotics, as well as implementing
improvements in infection control and optimizing medical practice. The
following table summarizes some of the infection control measures for
MRSA.
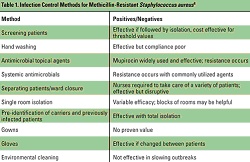
Table 1. Infection Control
Methods for Methicillin-Resistant Staphylococcus aureus4
Adapted from Haddadin AS, et al. Postgrad Med J. 2002;78:388, with
permission from BMJ Publishing Group Ltd. of the BMA. Copyright ©2002
BMJ Publishing Group Ltd. and the Fellowship of Postgraduate Medicine.
All rights reserved.
Hand Washing
Hand washing is widely
acknowledged to be the single most important activity for reducing the
transmission of infectious agents by both contact and fecal-oral
routes.7 Human skin can harbor large quantities and varieties of both
infectious and noninfectious microorganisms such as MRSA, which is
transmitted primarily on the hands of healthcare workers.8 But
compliance with hand hygiene protocols is generally less than optimal.
Healthcare workers tend to routinely wash their hands less frequently
and for a shorter duration than hospital policy dictates. Pittet et
al9 found that compliance with hand washing protocols averaged about
48% among health professionals in a teaching hospital. Noncompliance
was higher among physicians, nursing assistants, and other healthcare
workers than among nurses. Compliance with hand washing guidelines
also was higher in general medical wards than in the ICU and was lower
during procedures that carried a high risk for contamination (eg,
before intravenous care, 39%; before respiratory care, 18%).
Shortages of staffing and
higher patient workloads have contributed to poor compliance with hand
washing.6,7 Frequent and vigorous hand washing also can damage and
break the skin, causing changes in microbial flora and increasing the
risk of microorganism transmission.7 Alternative hand washing methods
using waterless alcohol-based products have been developed. These are
less harsh to the skin than soap and water, do not require access to a
sink, and have been shown to be superior to traditional hand washing
in reducing bacteria on skin surfaces.10 Voss et al11 found that full
compliance with guidelines may be problematic, as workers in the ICU
needed a full minute to walk to the sink, wash their hands, and then
return to the patient. But whereas between 40 and 80 seconds are
needed to wash with soap and water, cleaning the hands with an
alcohol-based solution takes only 20 seconds. Thus, compliance may
improve by increasing the use of alternative methods.
Using Antibiotics
Wisely
Although there is no
single approach that can eliminate drug-resistant pathogens, judicious
use of antibiotics is essential for improving patient outcomes,
providing better empiric therapy, and minimizing the chances of
further antibiotic resistance.
Treat Appropriately
Up-front
Adequate initial
antimicrobial treatment is generally defined as an antibiotic regimen
that has demonstrated in vitro activity against the pathogen
associated with an infection.12 Inadequate treatment has been
identified as an important factor in the emergence of infections due
to antibiotic-resistant bacteria.13
Increasing clinical
evidence suggests that failure to initially treat serious bacterial
infections such as hospital-acquired pneumonia and bacteremia with
appropriate antimicrobial agents is associated with an increase in
patient morbidity and mortality.13 In a study of patients with
ventilator-associated pneumonia (VAP), Luna et al 14 found that when
adequate antibiotic therapy is initiated very early, even before
performing a bronchoscopy, the mortality rate is reduced. But if
adequate therapy is delayed until diagnosis is confirmed by
bronchoscopy or bronchoalveolar lavage (BAL), then mortality is
higher. Even if the antimicrobial regimen is changed to one that is
more effective, the mortality rate remains comparable with that of
patients who continue to receive inadequate therapy.
The necessity of providing
adequate initial antimicrobial treatment needs to be balanced with
trying to limit the emergence of drug resistance.13 Patients at high
risk for infection with antibiotic-resistant bacteria need to be
identified. These risk factors include prior antibiotic exposure, use
of broad-spectrum antibiotics, prolonged length of hospital stay,
prolonged mechanical ventilation, and presence of invasive devices.
Healthcare-associated infections also are generally caused by
pathogens similar to those responsible for hospital-acquired
infections, and identification of these patients is essential to
avoiding initial inadequate treatment. Risk factors include receiving
home parenteral therapy, hemodialysis treatment at a clinic, recent
hospitalization, or residence in a nursing home or long-term care
facility. The treatment regimen then needs to be based on individual
patient characteristics as well as appropriately using the data
presented in an updated hospital-specific or unit-specific antibiogram.
Avoid Unnecessary
Therapy
Antibiotics are an
integral part of treating bacterial infections, but their use can be
minimized without compromising the patient. De-escalation, giving a
shorter course of treatment, and stopping antibiotics when an
infection is ruled out all balance the need to provide adequate
initial treatment to high-risk patients, while avoiding unnecessary
antibiotic use.13
The goal of de-escalation
is to first provide an adequate initial antibacterial regimen that
will most likely be effective against the bacterial pathogens
associated with infection and then to narrow the antibiotic spectrum
once the pathogens and their susceptibility profiles are identified.13
Because of the high mortality rates associated with delays in adequate
treatment,14 it is important to begin an empiric antimicrobial
treatment at the first sign of infection, especially in critically ill
patients. But in order to minimize the emergence of drug-resistant
infections, the antimicrobial regimen can be narrowed or discontinued
completely. This can usually be accomplished within 48 hours of
beginning treatment.15
Studies have shown that
antibiotic therapy can be shortened in specific patient populations
without having an adverse effect on patient outcome.16 Evans et al17
used a computerized guideline to determine antibiotic prescribing in
the ICU, and patients with computer-guided therapy had marked
reductions in the mean number of days of excessive drug dosage (2.7 vs
5.9) compared with those followed by clinicians not using the program.
Clinical outcomes were similar, but length of stay and costs were
lower for the patients guided by the computerized anti-infectives–management
program.
Taking another approach,
Singh et al18 found that antibiotic regimens could be both shortened
and discontinued in patients with suspected VAP by using the clinical
pulmonary infection score (CPIS) as a treatment guideline. Patients
with a CPIS of 6 or less, suggesting that they were less likely to
have pneumonia, were randomized to receive either standard therapy
(choice and duration of antibiotics determined by the clinician) or
ciprofloxacin monotherapy with reevaluation at 3 days (Figure 1
below). If the CPIS was still less than or equal to 6, antimicrobial
therapy was discontinued. Mortality and length of ICU stay were found
to be similar between the 2 groups, despite the shorter duration of
therapy. Patients on the shorter regimen also had fewer
superinfections with resistant pathogens, including a lower number of
infections due to MRSA and P. aeruginosa.
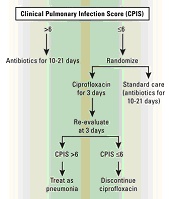
Figure 1. Clinical
Pulmonary Infection Score (CPIS)
Flow chart of Singh et al study protocol. Reproduced with permission
from Singh N, et al. Am J Respir Crit Care Med. 2000;162:506, with
permission from the American Thoracic Society. Official journal of the
American Thoracic Society. Copyright ©2000 American Thoracic Society.
All rights reserved.
Disease-Specific
Strategies
The most common
hospital-acquired infections are those of the urinary tract (UTI),
surgical site, respiratory tract, and bloodstream.19 In the ICU
setting, the presence of an invasive device is overwhelmingly
associated with a nosocomial infection; 83% of episodes of nosocomial
pneumonia were associated with mechanical ventilation, 97% of UTIs
occurred in catheterized patients, and 87% of primary bloodstream
infections occurred in patients with a central line.
Ventilator-Associated
Pneumonia
Pneumonia is one of the
most commonly reported nosocomial infections and is frequently
reported in patients receiving mechanical ventilation. Reported
prevalence of VAP ranges from 7% to 65%20,21 and has been associated
with increased morbidity, mortality, and a longer length of hospital
stay. The causative pathogens vary among healthcare facilities,
depending on the population of patients in the ICU, the durations of
hospital and ICU stays, and the specific method used to diagnose VAP.
A large percentage of VAP cases are increasingly caused by
gram-negative bacilli, with S aureus being the predominant
gram-positive isolate.21 Etiology also depends on whether the VAP is
early or late onset and if the patient has been previously treated
with antibiotics.
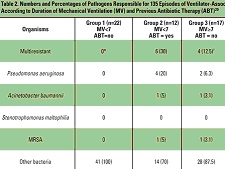
Table 2. Numbers and
Percentages of Pathogens Responsible for 135 Episodes of
Ventilator-Associated Pneumonia Classified According to Duration of
Mechanical Ventilation (MV) and Previous Antibiotic Therapy (ABT)20
* P<.02 versus Groups 2, 3, or 4.
† P<.0001 versus Group 4
Reproduced from Trouillet J-L, et al. Am J Respir Crit Care Med.
1998;157:534, with permission from the American Thoracic Society.
Official journal of the American Thoracic Society. Copyright ©1998
American Thoracic Society. All rights reserved.
Trouillet et al20 found
high rates of Haemophilus influenzae, Streptococcus pneumoniae,
methicillin-susceptible S aureus (MSSA), and susceptible
Enterobacteriaceae in patients with early-onset VAP, particularly in
those who had not received prior antimicrobial treatment (Table 2). In
contrast, potentially multidrug-resistant pathogens such as P
aeruginosa, Acinetobacter baumannii, Stenotrophomonas maltophilia, and
MRSA were the predominant organisms in patients with late-onset VAP
and who had recently been treated with antibiotics. Risk factors for
VAP caused by potentially drug-resistant bacteria were identified as
prior antibiotic use, prior use of broad-spectrum agents, and the
duration of mechanical ventilation before the onset of VAP. Lower
respiratory tract colonization by MRSA and other resistant organisms
also closely correlates with the subsequent development of
pneumonia.22
Strategies aimed at
reducing the burden of bacterial colonization in the aerodigestive
tract and decreasing the incidence of aspiration will help prevent the
onset of VAP, and similarly, strategies to reverse the trend of
resistance also can be implemented. Prolonged antibiotic
administration to ICU patients is believed to favor selection and
subsequent colonization with resistant pathogens.21 Prophylactic use
of parenteral broad-spectrum antibiotics is not recommended as it may
increase the risk of VAP caused by multiresistant pathogens, but only
delays and does not prevent the occurrence of nosocomial
infection.15,21
A gold standard for the
diagnosis of VAP does not exist, and as the clinical criteria for
pneumonia is nonspecific, inappropriate use of antibiotics for
pulmonary infiltrates is common in the ICU. Studies have documented
empiric antibiotic treatment for infiltrates in the absence of
pneumonia, which in turn raises the prospect of antimicrobial
resistance.18 The use of quantitative cultures obtained with BAL, and
quantitative assessments that determine the risk of VAP, have reduced
prolonged antibiotic use in many patients with suspected infection and
lowered both antimicrobial resistance and the cost of antimicrobial
treatment.14,22
Bacteremia
Bloodstream infections are
among the most serious infections acquired by hospitalized patients,
and antibiotic resistance appears to have contributed to an increasing
degree of inadequate antimicrobial therapy. In a prospective cohort
study of clinical outcomes of patients in the ICU, Ibrahim et al5
identified a statistically significant relationship between the rates
of inadequate antimicrobial treatment for individual pathogens and
their associated rates of mortality. The most common microorganisms
identified and their rates of inadequate antimicrobial treatment
included vancomycin (100%), Candida species (95.1%), oxacillin-resistant
S aureus (32.6%), coagulase-negative staphylococci (21.9%), and P
aeruginosa (10%). In a pooled analysis, Cosgrove and colleagues23 also
reported that MRSA bacteremia was associated with a significantly
higher mortality rate than bacteremia caused by MSSA.
A substantial number of
cases of bacteremia may be related to intravascular catheters. Over
200,000 bloodstream infections occur in the United States annually,
and most are related to an intravascular device, primarily the
nontunneled type.24 The risk factors for catheter-related infections
vary, according to location of catheter and duration of use and
hospital size, unit, or service. Strategies for reducing
catheter-related bacteremia include the use of full barrier
precautions during catheter insertion; subcutaneous tunneling of
short-term catheters inserted in the internal jugular or femoral veins
when catheters are not used for drawing blood; the use of
antimicrobial-impregnated catheters; povidone-iodine ointment applied
to insertion sites of hemodialysis catheters; no routine replacement
of central venous catheters; and contamination shields for pulmonary
artery catheters.25,26
The risk for infection
also has been shown to be higher with catheters inserted into the
internal jugular vein, compared with insertion into the subclavian
vein.25 In addition, other data indicate that femoral venous
catheterization is associated with a greater risk of both infection
and thrombosis.27
Urinary Tract
Infections
Urinary tract infections
are the most common nosocomial infection and are often persistent and
difficult to treat.19 The overwhelming majority are related to the
presence of an indwelling catheter, although other risk factors may
play a role. Although UTIs may often be asymptomatic and less
expensive to treat than other infections, they can be major reservoirs
of antimicrobial-resistant pathogens.
Indwelling urinary
catheters often have been unnecessarily used or continued longer than
necessary. In one study, the initial indication for the placement of
an indwelling catheter was found to be unjustified in 21% of 202
medical patients,28 and continued use was found to be unjustified in
47% of 912 patient-days. According to the Centers for Disease Control
and Prevention, catheters should only be used when essential and
removed when no longer needed.29 If a urinary catheter is deemed
necessary, proper aseptic technique, including insertion and
maintenance of the catheter and drainage bag, is essential in helping
to prevent a UTI.26,29
Surgical Site
Infections
About 700,000 surgical
site infections occur annually in the United States, making them the
most common nosocomial infection in surgical patients.30 While the
causative organisms may vary depending on the operative site, an
increasing proportion of infections are caused by resistant pathogens.
Methicillin-resistant S aureus alone now accounts for 28% of all
surgical wound infections.31 A study by Gleason et al32 observed that
of 441 surgical site infections caused by gram-positive cocci, 248
were antibiotic-resistant strains. Patients with infections caused by
the resistant organisms also had a poorer prognosis.
Surgical site infections
result from a variety of factors, including bacterial contamination of
the wound during the procedure, virulence of the contaminating
organisms, adjuvant factors within the surgical wound, and patient
risk factors. Use of prophylactic antibiotics is generally recommended
in curtailing the rates of wound infection, but they need to be
administered in a way that does not promote antimicrobial-resistant
bacteria. The general consensus is that a dose of an appropriate
antibiotic be administered within 60 minutes of the surgical incision
to ensure that adequate drug concentrations are present in the serum,
tissue, and wound during the entire time that the incision is open and
at risk for bacterial contamination.33 Prophylactic antimicrobials
should then be discontinued within 24 hours after completion of the
procedure, except for cardiothoracic surgery.
Nasal colonization with S
aureus may be an important independent risk factor for developing a
subsequent surgical site infection. Kluytmans and colleagues34 studied
the perioperative elimination of nasal carriage using mupirocin nasal
ointment. Investigators reported that a significant reduction in the
infection rate following cardiothoracic surgery was observed in
patients who had been treated with mupirocin compared with those who
were not (2.8% vs 7.3%, respectively).
Optimization of
Antimicrobial Therapy
The efficacy of
antimicrobial therapy depends upon a number of factors, including the
ability to penetrate tissue and the degree to which it binds with
protein, which varies considerably among agents. Pharmacokinetic
parameters, physicochemical characteristics, and extravascular
distribution all play a role in how well an antibiotic will be able to
kill or disable the targeted pathogen.35 Physicochemical
characteristics may differ between drugs of the same class, such as in
the case of fluoroquinolones, which have a high degree of
liposolubility. For example, ciprofloxacin is hydrophilic, whereas
sparfloxacin is lipophilic, and ofloxacin and lomefloxacin exhibit
intermediate liposolubility. However, all agents in this class are
similar as far as their tissue diffusion characteristics.
After administration, the
antimicrobial reaches a stable level in the plasma and eventually
binds with plasma proteins. It is then gradually distributed
throughout body tissues and fluids. The most appropriate method of
antimicrobial administration needs to be determined to promote optimal
efficacy. In one study that compared a continuous infusion of
ceftazidime with intermittent administration, investigators reported
that the continuous infusion was more consistent in keeping serum
concentrations of the drug above minimal inhibitory concentrations (MICs)
of 4 µg/mL.36 Both methods achieved the same pharmacodynamic
parameters, but the continuous infusion used only half the daily dose
of intermittent administrations. A continuous infusion offers the
potential for lower antimicrobial doses and institutional cost
savings.
The ability of an
antibiotic to concentrate at the infection site is an important factor
in determining the success of therapy.35 For example, in UTIs renal
tissue concentrations of an antibiotic are a good indicator of
efficacy.37 The degree of protein binding is a strong determinant of
both the agent's half-life and tissue penetration. Antibiotics with a
high affinity to bind with protein tend to have long serum half-lives,
whereas low protein-binding agents have short ones.38 Agents that are
highly protein bound, such as vancomycin and ceftriaxone, also do not
disperse as readily into extravascular sites such as the lungs, bone,
and muscle.35,38
Although vancomycin has
historically been the treatment of choice for MRSA infections, it may
not be the best option for pneumonia. Data from 2 prospective,
randomized, double-blind studies that compared linezolid with
vancomycin in the treatment of documented pneumonia caused by S aureus,
as well as a subset of patients with MRSA pneumonia, indicated that
vancomycin was a less effective treatment.39 Patients in the MRSA
subset who were treated with linezolid had better survival (80.0% vs
63.5%, P=.03) and clinical cure rates (59.0% vs 35.5%, P<.01) than
those who received vancomycin (Figure 2 below). The reason for
improved survival in the linezolid group may be due to the poor
penetration of vancomycin into the lungs, which has been documented in
other studies. Cruciani et al40 evaluated lung tissue penetration of
vancomycin in 30 patients undergoing lung resection. Concentrations of
vancomycin were undetectable in lung tissue in 1 of 6 patients
observed after 6 hours and in 3 of 7 patients at 12 hours. Another
investigation that studied vancomycin penetration in epithelial lining
fluid indicated that after being administered intravenously at a daily
dose of 30 mg/kg, drug levels were undetectable in 6 of 10 patients.41
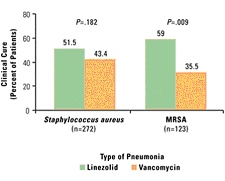
Figure 2. Type of
Pneumonia
Clinical cure rates for linezolid and vancomycin therapy in patients
with gram-positive nosocomial pneumonia. Reproduced from Wunderink RG,
et al. Chest. 2003;124:1795, with permission from the American College
of Chest Physicians. Copyright ®2003 the American College of Chest
Physicians. All rights reserved.
Toxin Production
The ability to produce
toxins is an underlying mechanism harbored by many bacterial
pathogens. These include S aureus alpha-toxin, Shiga toxin, cytotoxic
necrotizing factor type 1, Escherichia coli heat-stable toxin,
botulinum and tetanus neurotoxins, and S aureus toxic shock syndrome
toxin. The toxins are not susceptible to antibiotics, and destroying
the pathogen before too much toxin is produced often is the only
approach to preventing toxic effects, as antitoxins do not exist for
many of the bacterial toxins. But at subinhibitory concentrations,
antibiotics whose mode of action comprises inhibition of bacterial
protein biosynthesis also may modulate the expression of bacterial
virulence factors. At sub-MIC concentrations, clindamycin failed to
inhibit growth of Streptococcus pyogenes but modified its virulence.42
In a more recent study, subgrowth-inhibitory concentrations of
linezolid reduced virulence factors of S aureus and S pyogenes.43
Ohlsen et al44 used a gene
fusion model to evaluate the effects of 31 antibiotics on the
expression of alpha-toxin (hla) in both MSSA and MRSA isolates at
concentrations below the MIC. Glycopeptide antibiotics had virtually
no effect on the toxin, whereas erythromycin and several of the
aminoglycosides reduced its expression, and fluoroquinolones slightly
stimulated hla expression. But the main findings of the study were
that beta-lactams strongly induced hla expression; clindamycin almost
completely represses hla expression; and methicillin enhances hla
production of both MSSA and MRSA. Low concentrations of clindamycin
also have been shown to inhibit the expression of staphylococcal toxic
shock syndrome toxin.
Conclusion
Preventing
antibiotic-resistant infection requires a multifaceted approach.
Judicious use of antimicrobials is key to preventing the emergence of
further resistance, as well as implementation of comprehensive
infection control programs. Antibiograms need to be updated regularly
to keep track of bacterial resistance patterns, and clinicians also
need to be aware of which pathogens are primarily responsible for both
community-acquired and nosocomial infections. Strategies for
preventing infection, such as minimizing the use of invasive devices
and reducing bacterial colonization, will not only reduce the rate of
infections but also improve patient outcomes, reduce the prevalence of
antimicrobial-resistant pathogens, and decrease healthcare-related
costs.
The antibiotic
susceptibility profiles of these pathogens should be routinely
available to physicians to guide their selection of antimicrobials,
particularly as few new classes of agents are likely to become
available for clinical use in the short term. Preventing
healthcare-associated infections caused by antimicrobial-resistant
pathogens requires a comprehensive approach that includes: (1)
preventing infections through the use of vaccines and prophylaxis; (2)
minimizing the use of invasive devices; (3) understanding,
implementing, and complying with current guideline recommendations for
the prevention of infections; and (4) using antimicrobials
judiciously. Implementing such a comprehensive program will reduce
healthcare-associated infections, reduce the prevalence of
antimicrobial-resistant pathogens, reduce healthcare costs, and
improve patient outcomes.
Finally, there is dire
need for continued development of new antibiotics and new technologies
to improve their delivery to the infection site. Continued research is
needed to identify and develop new and innovative approaches to
treating infectious diseases.
References
-
Centers for Disease Control and Prevention. Prevent Antimicrobial
Resistance in Healthcare Settings. Available at:
http://www.cdc.gov/drugresistance/healthcare/problem.htm.
Accessed December 12, 2004.
-
Centers for Disease Control and Prevention. Selected antimicrobial
resistant pathogens associated with nosocomial infections in ICU
patients, comparison of resistance rates from January-December 1999
with 1994-1998, NNIS System. Available at:
http://www.cdc.gov/ncidod/hip/NNIS/ar_surv99.pdf. Accessed
December 12, 2004.
-
Hanberger H, Garcia-Rodriguez JA, Gobernado M, et al. Antibiotic
susceptibility among aerobic gram-negative bacilli in intensive care
units in 5 European countries. JAMA. 1999;281:67-77.
-
Haddadin AS, Fappiano SA, Lipsett PA. Methicillin resistant
Staphylococcus aureus (MRSA) in the intensive care unit. Postgrad
Med J. 2002;78:385-392.
-
Ibrahim EH, Sherman G, Ward S, et al. The influence of inadequate
antimicrobial treatment of bloodstream infections on patient
outcomes in the ICU setting. Chest. 2000;118:146-155.
-
Kollef MH, Fraser VJ. Antibiotic resistance in the intensive care
unit. Ann Intern Med. 2001;134:298-314.
-
Larson E. Skin hygiene and infection prevention: more of the same or
different approaches? Clin Infect Dis. 1999;29:1287-1294.
-
Boyce
JM. MRSA patients: proven methods to treat colonization and
infection. J Hosp Infect. 2001;48(Suppl A):S9-S14.
-
Pittet D, Mourouga P, Perneger TV. Compliance with handwashing in a
teaching hospital. Infection Control Program. Ann Intern Med.
1999;130:126-130.
-
Zaragoza M, Salles M, Gomez J, et al. Handwashing with soap or
alcoholic solutions? a randomized clinical trial of its
effectiveness. Am J Infect Control. 1999;27:258-261.
-
Voss
A, Widmer AF. No time for handwashing!? handwashing versus alcoholic
rub: can we afford 100% compliance? Infect Control Hosp Epidemiol.
1997;18:205-208.
-
Iregui M, Ward S, Sherman G, et al. Clinical importance of delays in
the initiation of appropriate antibiotic treatment for
ventilator-associated pneumonia. Chest. 2002;122:262-268.
-
Kollef M. Inadequate antimicrobial treatment: an important
determinant of outcome for hospitalized patients. Clin Infect Dis.
2000;31:S131-S138.
-
Luna
CM, Vujacich P, Niederman MS, et al. Impact of BAL data on the
therapy and outcome of ventilator-associated pneumonia. Chest.
1997;111:676-685.
-
Kollef MH, Sherman G, Ward S, Fraser VJ. Inadequate antimicrobial
treatment of infections: a risk factor for hospital mortality among
critically ill patients. Chest. 1999;115:462-474.
-
Kollef MH. An empirical approach to the treatment of multidrug-resistant
ventilator-associated pneumonia. Clin Infect Dis. 2003;36:119-1121.
-
Evans
RS, Pestotnik SL, Classen DC, et al. A computer-assisted management
program for antibiotics and other antiinfective agents. N Engl J
Med. 1998;338:232-238.
-
Singh
N, Rogers P, Atwood CW, et al. Short-course empiric antibiotic
therapy for patients with pulmonary infiltrates in the intensive
care unit: a proposed solution for indiscriminate antibiotic
prescription. Am J Respir Crit Care Med. 2000;162:505-511.
-
Richards MJ, Edwards JR, Culver DH, Gaynes RP. Nosocomial infections
in combined medical-surgical intensive care units in the United
States. Infect Control Hosp Epidemiol. 2000;21:510-515.
-
Trouillet JL, Chastre J, Vuagnat A, et al. Ventilator-associated
pneumonia caused by potentially drug-resistant bacteria. Am J Respir
Crit Care Med. 1998;157:531-539.
-
Chastre J, Fagon JY. Ventilator-associated pneumonia. Am J Respir
Crit Care Med. 2002;165:867-903.
-
Kollef MH. The prevention of ventilator-associated pneumonia. N Engl
J Med. 1999;340:627-634.
-
Cosgrove SE, Sakoulas G, Perencevich EN, et al. Comparison of
mortality associated with methicillin-resistant and methicillin-susceptible
Staphylococcus aureus bacteremia: a meta-analysis. Clin Infect Dis.
2003;36:53-59.
-
Mermel LA, Farr BM, Sherertz RJ, et al. Guidelines for the
management of intravascular catheter-related Infections. Clin Infect
Dis. 2001;32:1248-1272.
-
Mermel LA. Prevention of intravascular catheter-related infections.
Ann Intern Med. 2000;132:391-402.
-
Saint
S, Savel RH, Matthay MA. Enhancing the safety of critically ill
patients by reducing urinary and central venous catheter-related
infections. Am J Respir Crit Care Med. 2002;165:1475-1479.
-
Merrer J, De Jonghe B, Golliot F, et al, and the French Catheter
Study Group in Intensive Care. Complications of femoral and
subclavian venous catheterization in critically ill patients: a
randomized controlled trial. JAMA. 2001;286:700-707.
-
Jain
P, Parada JP, David A, Smith LG. Overuse of the indwelling urinary
tract catheter in hospitalized medical patients. Arch Intern Med.
1995;155:1425-1431.
-
Centers for Disease Control and Prevention. CDC Campaign to Prevent
Antimicrobial Resistance in Healthcare Settings: 12 Steps to Prevent
Antimicrobial Resistance Among Hospitalized Adults. Available at:
http://www.cdc.gov/drugresistance/healthcare/patients.htm.
Accessed December 12, 2004.
-
Nathens AB, Dellinger EP. Surgical site infections. Curr Treatment
Options Infect Dis. 2000;2:347-358.
-
Baquero F. Gram-positive resistance: challenge for the development
of new antibiotics. J Antimicrob Chemother. 1997;39(Suppl A):1-6.
-
Gleason TG, Crabtree TD, Pelletier SJ, et al. Prediction of poorer
prognosis by infection with antibiotic-resistant gram-positive cocci
than by infection with antibiotic-sensitive strains. Arch Surg.
1999;134:1033-1040.
-
Bratzler DW, Houck PM. Antimicrobial prophylaxis for surgery: an
advisory statement from the National Surgical Infection Prevention
Project. Clin Infect Dis. 2004;38:1706-1715.
-
Kluytmans JA, Mouton JW, VandenBergh MF. Reduction of surgical-site
infections in cardiothoracic surgery by elimination of nasal
carriage of Staphylococcus aureus. Infect Control Hosp Epidemiol.
1996;17:775-779.
-
Bergogne-Berezin E. New concepts in the pulmonary disposition of
antibiotics. Pulm Pharmacol. 1995;8:65-68.
-
Benko
AS, Cappelletty DM, Kruse JA, Rybak MJ. Continuous infusion versus
intermittent administration of ceftazidime in critically ill
patients with suspected gram-negative infections. Antimicrob Agents
Chemother. 1996;40:691-695.
-
Honeybourne D, Baldwin DR. The site concentrations of antimicrobial
agents in the lung. J Antimicrob Chemother. 1992;30:249-260.
-
Stevens DL. The Relevance of Antibiotic Tissue Penetration for
Treating Complicated Skin and Soft Tissue Infections Caused by
Methicillin-Resistant Staphylococcus aureus: An Evidence-Based
Review. Tampa, Fla: MedScholar; 2004.
-
Wunderink RG, Rello J, Cammarata SK, et al. Linezolid vs vancomycin:
analysis of two double-blind studies of patients with methicillin-resistant
Staphylococcus aureus nosocomial pneumonia. Chest.
2003;24:1789-1797.
-
Cruciani M, G Gatti, Lazzarini L, et al. Penetration of vancomycin
into human lung tissue. J Antimicrob Chemother. 1996;38:865-869.
-
Georges H, Leroy O, Alfandari S, et al. Pulmonary disposition of
vancomycin in critically ill patients. Eur J Clin Microbiol Infect
Dis. 1997;16:385-388.
-
Gemmel CG, Peterson PK, Schmeling D. Potentiation of opsonization
and phagocytosis of Streptococcus pyogenes following growth in the
presence of clindamycin. J Clin Invest. 1981;67:1249-1256.
-
Gemmel CG, Ford CW. Virulence factor expression by Gram-positive
cocci exposed to subinhibitory concentrations of linezolid. J
Antimicrob Chemother. 2002;50:665-672.
-
Ohlsen K, Ziebuhr W, Koller KP, et al. Effects of subinhibitory
concentrations of antibiotics on alpha-toxin (hla) gene expression
of methicillin-sensitive and methicillin-resistant Staphylococcus
aureus isolates. Antimicrob Agents Chemother. 1998;42:2817-2823.
|